Fuel cells have the capacity to be the most effective and ecologically friendly power source ever devised. Since fuel cells are able to generate drinking water as a by-product, they are indeed an appealing replacement for traditional power generation. How much more could you want from a source of energy?
History
Sir William Grove developed the theory of a working fuel cell in the year 1839. He discovered that feeding hydrogen and oxygen to two different electrodes submerged in sulfuric acid could create electricity. Fuel cells, on the other hand, continued to be a mystery for more than a century.
Because of technological and economic barriers, the idea of fuel cell functioning has eluded practical applicability for a long time. It wasn't until the 1960s, with the expansion of the space exploration, the goal to develop fuel cell technology as a sustainable power substitute for conventional power generation reignited.
Fuel Cells vs Conventional Power Generators
Efficacy and pollution are two major challenges in traditional power generation. The majority of the world's electricity is generated by steam turbines that use the heat created by the combustion of coal.
Mechanical systems have a lot of energy conversion steps, and the rules of thermodynamics restrict their efficiency. As a result, there are significant power wastage. In contrast, a fuel cell turns the inherent chemical energy of fuel into electricity.
Since it runs at a specific temperature during the electrochemical process, it is not constrained by the thermodynamic principles that limit heat engines.
Combustion, industrial processes, and vehicle emissions all contribute to air pollution. Incomplete combustion of fuel, partially burnt fuel, carbon, hydrogen sulfide, co2, dust, sulphur dioxide, nitrogen oxides, and other toxins are among them.
Warming rivers due to surplus heat from power generation disrupts the natural balance of fish and fauna. And we're all aware of the devastation caused by acid rain, which is caused by man-made sulphur and nitrogen emission into the atmosphere.
In contrast, we have clean water as the by-product in a fuel-cell process. Who could argue with that?
Chemical Reactions in a Fuel Cell
In a flameless, catalytic process, fuel cells transform the energy stored in specific chemical interactions directly into electrical current. Around room temperature, several forms of fuel cells perform excellently.
An anode (+) and cathode (-) electrodes are separated by a conductive electrolyte, for example a potassium hydroxide solution, in a conventional fuel cell. The negative electrode accumulates the fuel, like hydrogen gas or hydrazine, which is oxidized generating electrons to the load.
The method of separating one or more electrons from an ion or molecule is known as oxidation. Electrochemical oxidation of the fuel produces hydrogen ions on the anode electrodes in fuel cells. If the fuel is hydrogen, the ionization process produces hydrogen ions, which can be expressed through the following formula:
H2 → 2H+ + 2e
Oxygen, air, or hydrogen peroxide (an oxygen resource) is delivered to the cathode electrode, where it is reduced, resulting in the splitting of the O2 oxygen molecule. During this process ionic conduction within the electrolyte causes the circuit to be completed. As seen in the following chemical equation, hydrogen and oxygen combine to generate water:
2 H2 + O2 → 2H2O
When hydrazine is oxidized, it produces nitrogen, which is a natural component of air and therefore is also safe:
N2H4+ O2 → 2H2O + N2
You would think that because hydrogen is such a "green" fuel, we can simply ignite it in atmosphere and produce pure water as a byproduct, along with electricity.
Burning hydrogen instead of coal, oil, or gasoline might be a significant improvement, but despite of this when air is burnt, a substantial quantity of nitrogen is sucked into the combustion process and burned at around 1000 degrees Celsius.
It partly interacts with oxygen at this high temperature, generating nitrogen oxides. Therefore, even though the primary product of this reaction is clean drinking water, the secondary reaction which produces nitrogen dioxide ruins it all by rendering the water unfit to drink.
This difficulty is solved when the reaction of hydrogen and oxygen is implemented at room temperature in a fuel cell.
Experimental Prototype
The approach described below can be used to build a good prototype fuel cell. But, we have to offer the following warning: This item should never be built or experimented with in any manner unless that's under the close supervision of an extremely capable chemist or chemical engineer. In a fuel cell, several chemicals and gaseous by-products might be poisonous and/or catastrophic!
As seen in Fig. 1 below, the proposed practical fuel cell has two adjacent compartments divided by a membrane. For each chamber, an catalytically active electrode is inserted. A liquid electrolyte is introduced across both chambers.
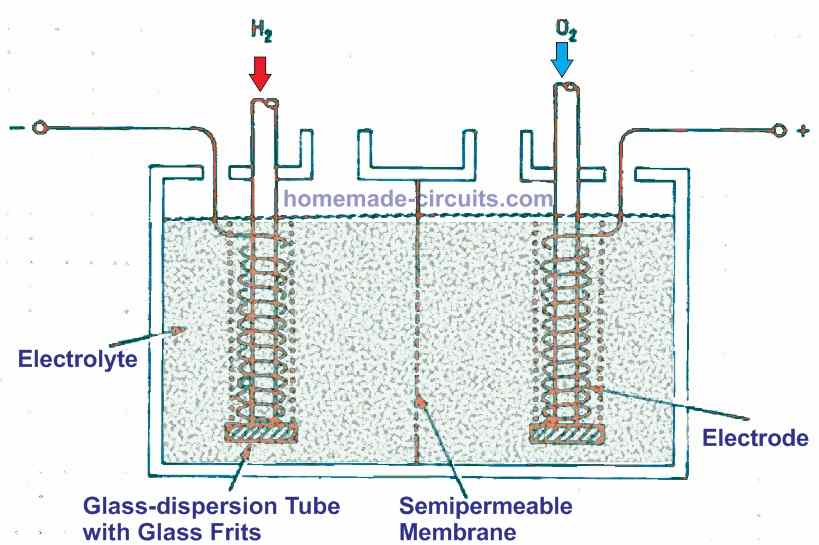
The hydrogen gas is then expelled from one electrode, while oxygen or air is expelled from the other, and a voltmeter is attached across the electrodes.
Semipermeable membranes and catalysts are required for the construction of a fuel cell. Just a few ions can travel through it, but it retains the rest of the substance. Ion segregation isn't really efficient in real-world applications, and some leakage is common and acceptable.
Absolute blockage, on the other hand, would stop a process.
Semipermeable membranes could be constructed from the following materials:
Baked clay discs without glaze (from an old clay flower pot). Frits of fine glass (the partly fused mixture of sand and fluxes which glass is made of). Cellophane. Plaster in wet condition. Cement that is damp and cured. Cement made with zinc oxide or zinc chloride. Plastic foam of a certain composition. Silicic acid gel made by acidifying sodium silicate solution gradually. Gelatin that has been soaked with salt.
Discs made of clay, cement, or plaster must be as thin as feasible. As per Fig. 2 below, the gels must be utilized to create ion bridges. Glass frits are available at lab supply stores and are ideal for this application. The gases rise higher and stay in the correct position when glass frits are utilized.
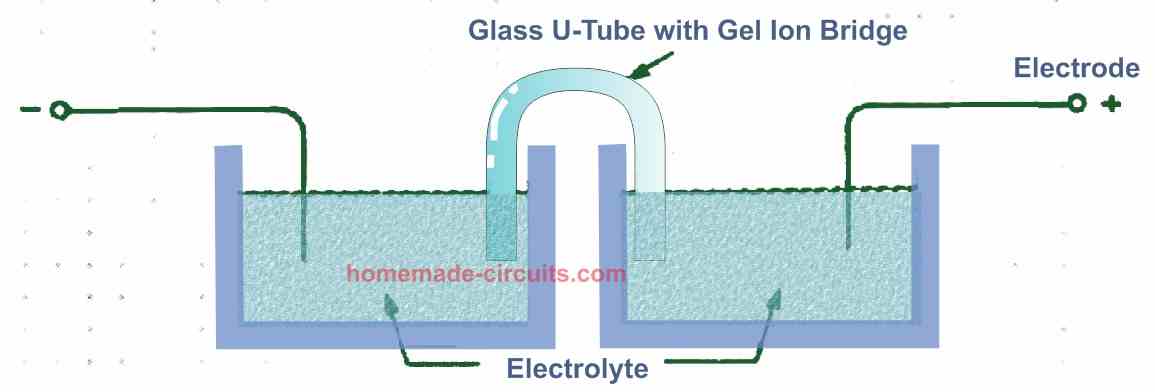
Any reasonable separation will suffice.
A couple of square polyethylene bottles were utilized for the prototype, along with a large fine glass frit that was cemented into holes punched in the bottle's sides, as indicated in (Fig. 3) below.
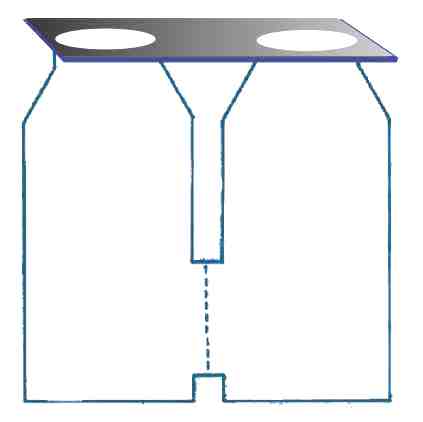
It takes a little coaxing to get hydrogen and oxygen to interact at ambient temperature. You won't find anything happening until the right catalyst is provided.
A catalyst is a substance that speeds up processes without actively participating in them. You won't find any electrical energy created if you configure an H2/O2 fuel cell using sulfuric acid and carbon electrodes, for example. The reaction begins only when platinum or palladium-coated carbon electrodes are utilized.
Wrapping platinum wire and a platinum mesh all around carbon rods is one of the methods for plating carbon, and it works quite well. Coating nickel mesh with palladium is a simple and inexpensive approach to produce a huge surface of palladium.
This may be accomplished by soaking a nickel net overnight in a palladium dichloride solution of 2%. The finishing appears to be black. Palladium-coated nickel has the same properties as pure palladium. Unless platinum is accessible to you, you would want to go with the method described above.
A coil of electrodes is wrapped around the tube. Leads made of copper wire are interconnected. A 30 percent potassium hydroxide solution serves well as the electrolyte. Laboratories containers of oxygen and hydrogen with relatively cheap lab-reduction valves could be purchased.
Zinc and diluted hydro-chloric acid could also be used to make hydrogen. This leaves you with a zinc chloride solution that is dangerous to the environment and should be disposed of according to regulations.
In order for the flammable hydrogen to escape, the complete experiment was done outside in the open air for 12 hours. Gas flow was measured using rotameters. If you like, bubble indicators could be used instead. The gas flow rate was 10-20 litres per hour (1/h), however it could be varied. The flow of oxygen should be around half that of hydrogen. Because hydrogen must saturate the platinum metal surface, the reaction process can be slow initially.
For many minutes, a reading of roughly 10 mV may be seen, which will gradually increase. Because there may be stages in this increase, a small drain can be introduced by connecting a 100-ohm resistor between the two chambers. After some minutes, it may be withdrawn again. This aids in the reduction of polarisation effects. After around 10 minutes, the discussed prototype showed 998 mV.
The cell will create a voltage for a period of time after the hydrogen is switched off to adjust for the delayed start. After you've finished, put the potassium hydroxide solution into a plastic bottle with a tight lid. It could be reused, although it will collect carbonate with time, making it less effective.
For the very same objective, some people prefer diluted sulfuric acid since it lasts longer. In most circumstances, air may be used instead of oxygen. However, because oxygen makes up just 1/5 of the air, the proportion must be increased. Although hydrogen peroxide could be substituted for oxygen, it might cause dilution of the electrolyte.
Hydrogen can be substituted by gases having hydrogen content such as "city gas," which contains hydrogen, methane, and carbon monoxide and is generated from coal. The fuel cell could also be utilized as a single-use unit for liquid fuels such as hydrazine and 30% hydrogen peroxide.
Both chemicals are usually used as rocket fuels, but these can be easily managed. They are, still nonetheless, extremely dangerous and toxic. Since hydrazine is a proven carcinogen, it must only be handled by those who are experienced and know how to work with extremely hazardous compounds. Hydrogen peroxide at a concentration of 30% can bleach your hands and must be administered with great caution.
You may be confused as to why fuel cells aren't more frequently used. The first major disadvantage is cost, which is mostly a major factor in power generation. Hydrogen is a costly fuel when compared to other forms of fuels, and storing it safely remains an issue.
Maybe in the near future, we'll be able to convert water into hydrogen and oxygen using solar energy on a big scale, which could then be stored. In a fuel cell, the two gases could be recombined into water whenever power is needed.
Post your comments here and get guaranteed replies. Comments must be related to the above article.