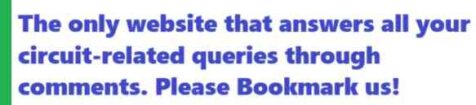
In this post we learn some basic facts about DC to DC buck converters and also learn how to build a simple buck converter circuit. A buck converter is also popularly known as a step-down converter, since its basic function is to step down a higher input voltage level to a lower output voltage level, with high efficiency.
The practical buck converter circuit explained here uses only 3 transistors and is extremely easy to build. Even though the circuit is simple, it has a high efficiency.
The circuit can be used to drive 3.3 V LEDs from a higher input supplies such as from 12 V, or 9 V supply inputs.
The buck converter design can be also easily upgraded to operate higher rated loads instead of an LED.
Advantages of Buck Converters Compared to Linear Regulators
The main advantage of a DC to DC buck converter compared to a linear regulator is that buck converters are hugely efficient than linear regulators.
Linear regulators such as IC 7812, IC 7805, LM317, LM338 waste a lot of energy through heat dissipation, whereas heat dissipation in buck converters is minimal.
Due to minimal heat dissipation, a heatsink may not be required in buck converters, or this may be very small in size.
The efficiency of linear regulators may range between 25% and 50%, the efficiency of buck converters is mostly around 90%.
Basic Working of a Buck Converter Topology
Referring to the figure below, let's try to understand how a 'buck' or a 'step-down' converter works. With a buck converter circuit, a higher input voltage can be transformed into a lower output voltage. Its basic mode of operation is described as follows.
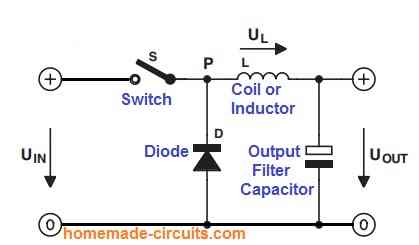

As soon as switch S is pressed, a positive voltage develops across the inductor L. This is because UIN is higher than Uout. The coil initially tries to resist the instantaneous current flow. As a result, the current in the coil increases linearly, and energy begins storing in the coil.
Next, as soon as switch S is opened, the stored current flows through the coil into the output capacitor through diode D.
Since the voltage UL across the coil is now negative, the current through the coil reduces linearly. The output receives the energy that was captured and stored in the coil. Now, if Switch S is closed once again, the procedure begins afresh and keeps repeating as the switch is operated ON/OFF.
Modes of Operation
The voltage that appears at the output is determined by how the switch S is operated. According to figure below, there are three basic types of current flow.
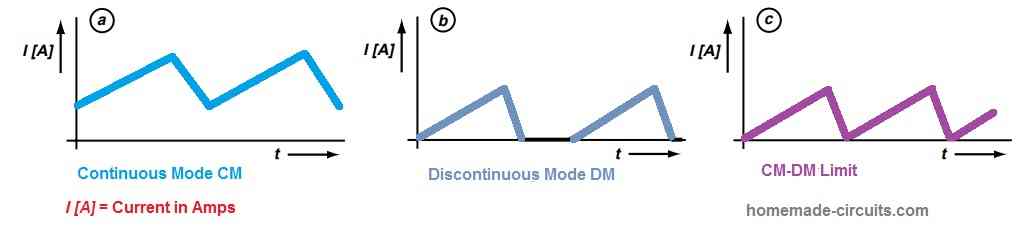
- Referring to the figure (a) above suppose, the switch S is closed and opened such that the current flowing inside the coil isn't able to reach zero. In this situation a constant flow of current will be experienced through the coil. This is referred to as "continuous mode" (CM).
- Now let's imagine the switch S is closed and opened such that current is able to reach zero for part of the cycle, as illustrated in Figure (b). Then the circuit can be assumed to be operating in 'discontinuous mode' (DM).
- In the third scenario if suppose the switch is closed and opened such that the coil current is able to exactly reach zero as shown in figure (c), then we call this as CM/DM limit operation.
This means that, in a buck converter both the output voltage and power could be varied by adjusting the "on" periods of the switch. This is also called the mark-space ratio.
That's enough theory; now let's examine a straightforward real-world circuit.
Making a Practical Buck Converter Design
The following figure shows a simple practical DC to DC buck converter circuit using only 3 transistors and a few other passive elements.
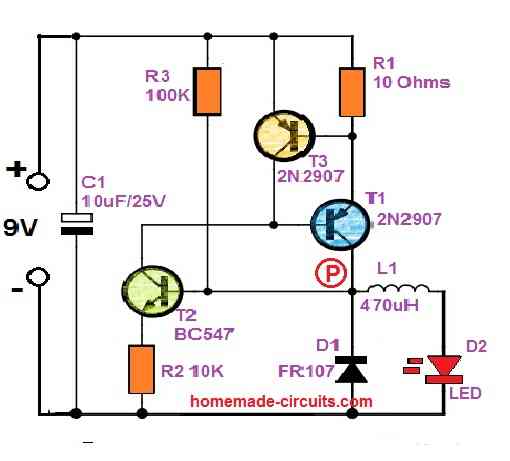
It works in the following manner:
Switch S in this circuit is represented by transistor T1. The other components of the step-down converter are diode D1 and coil L1.
As soon as the circuit is powered, R3 supplies a base current to T2 (because D2's forward voltage spec is larger than 0.7 V) and T2 is switched ON.
With T2 conducting, T1 gets a base bias and it also begins conducting. In this situation, point P experiences an increase in voltage, which causes T2 to conduct even harder.
Now as point P's voltage reaches 9 V, the current through L1 starts to increase. The voltage across the coil and its inductance both influence how quickly the current inside it increases.
As the current across the coil increases, the voltage across R1 decreases. As soon as this potential hits 0.7 V (about 70 mA) causes T3 to turn ON. This quickly removes the base current of T1.
Since the current in L1 can now no longer increase, the voltage at point P starts to decrease. T2 as a result is switched off, followed by T1.
The current via L1 now travels via D1 until it drops to zero. This causes the voltage on T2 to increase again, and the process repeats afresh.
The transistors work as a thyristor with positive feedback, resulting in an oscillation. T3 makes sure that T1 is shut off at the predetermined current and that the circuit operates in CM/DM limit mode.
Upgrading the Circuit for Higher Loads
Instead of illuminating an LED, you could employ this circuit to operate a higher rated load. But with a higher load you will find the buck converter not oscillating.
This is due to the load preventing R3 from turning on T2 at startup.
This issue may be avoided by placing a capacitor (0.1uF) between point P and the base of T2.
Another smart move would be to smooth the voltage by connecting a 10 F electrolytic capacitor across the output.
The buck converter functions as a current source instead of a voltage source and is unregulated. However, for most straightforward applications, this will be more than sufficient.
Simple Two Transistor Buck Converter Circuit
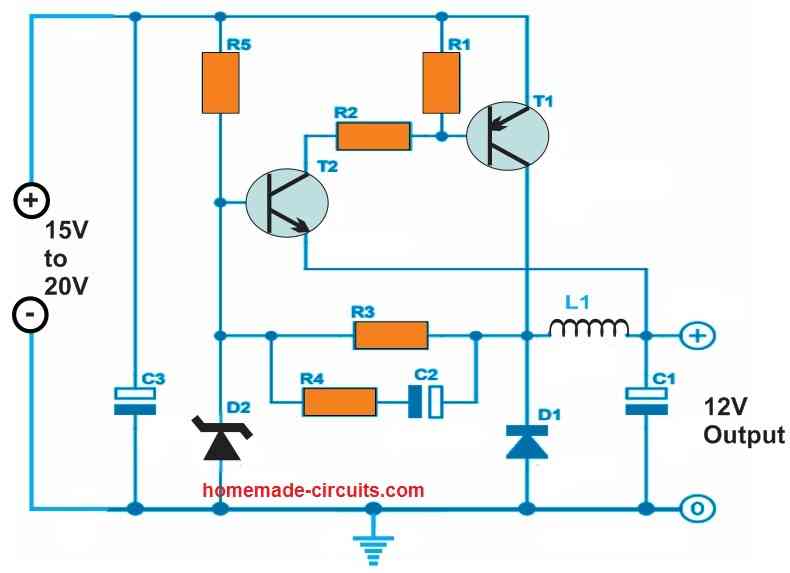
Parts List
- All resistors are 1/4 watt 5%
- R1 = 470 Ohms
- R2, R4 = 100 Ohms
- R3, R5 = 2.2 K
- Capacitors
- C1, C3 = 1000 uF/ 25 V
- C2 = 220 uF / 25 V
- Semiconductors
- D1 = FR107
- D2 = 12 V 1 watt Zener diode
- T1 = 2N2907
- T2 = BC547
- L1 = 120 uH
We can make the above design much simpler using just two transistors? Referring to the circuit diagram above, we can see a regulated step-down converter, which can generate 12 V DC from a 20 V DC. The main components of this design are transistor T1, diode D1 and coil L1.
The circuit works using the feedback between a combination of the PNP/NPN transistors. Here, the working of T1 is not dependent on the maximum coil current. Instead it is determined by the RC network of R4/C2.
The voltage regulation for this simple buck converter circuit is determined by the zener diode D2, associated with the emitter of T2.
This DC to DC buck converter design is highly efficient, which may be as high as 90%.
How to Build
- Step#1: Take 20 mm by 20 mm general purpose strip board.
- Spep#2: Clean the copper side with a sand paper.
- Step#3: Take the resistors and the diodes and bend their leads leaving 1 mm distance between their body and the leads.
- Step#4: Insert the resistors into the PCB and solder them. Cut the excess lead lengths.
- Step#5: Insert the transistors as per the same layout position as indicated in the schematic. Solder their leads, and trim the extended leads.
- Step#6: Now, insert the inductor, solder it, and trim its leads.
- Step#7: Finally insert, the capacitor and the LED, solder the leads. Cut the excess leads
Once the above assembly is done carefully interconnect the leads of the various components by referring to the schematic diagram. Do this using the pieces of the trimmed lead wires, previously cut.
If you are unable to connect the leads directly from the copper side, you can use jumper wire from the component side of the PCB.
How to Test
- Keep the LED disconnected at the start.
- Apply 9 V DC to the circuit.
- Measure the voltage across the points where the LED is supposed to be connected.
- It must be around 3 V to 4 V.
- This will confirm that you have build the buck converter correctly, and it is working correctly.
- You can switch OFF power and connect the LED back in its position.
- Now switch ON the DC again, you will find the LED illuminated brightly from the 9 V DC input with maximum efficiency.
How to Measure the Efficiency
To measure the efficiency you can connect an ammeter in series with the positive line of the 9 V DC.
Then you can multiply the current reading with the voltage (9 V).
The result could be somewhere around 20% higher than the watt specification of the LED.
This might prove an 80% efficiency for the buck converter circuit, and likewise.
Adjustable PWM Buck Converter Circuit
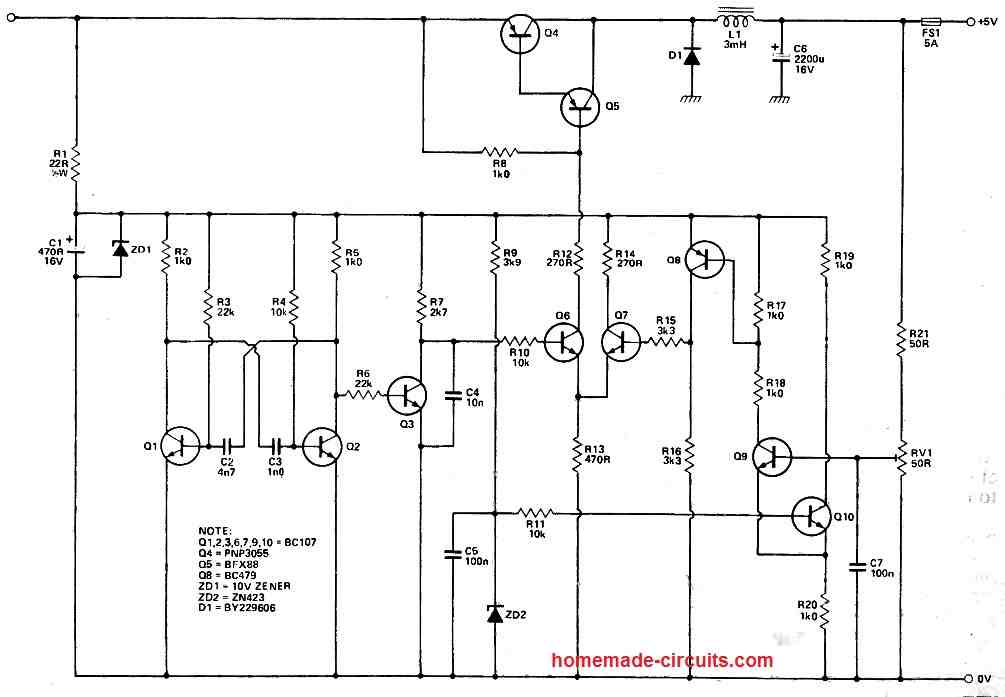
This circuit was initially designed with the purpose of generating a 5V power supply from a car battery to operate a BBC microcomputer.
Notably, the design refrains from employing integrated circuits (ICs), utilizing instead readily available discrete semiconductors salvaged from miscellaneous parts.
Efficiency Considerations
Achieving efficiencies surpassing 80%, this circuit outperforms linear regulators which generally exhibit maximum efficiencies ranging from 33% to 42%.
Astable Multivibrator and Pulse Generation
The operation commences with transistors Q1 and Q2 configured in an astable multivibrator arrangement.
This configuration yields a brief positive pulse at the base of Q3 at intervals of 50µs.
Consequently, the capacitor C4 discharges, subsequently recharging through the 2.7 kΩ resistor. This process delivers a gradually ascending voltage to the base of Q6.
Voltage Comparison and Regulation
Transistors Q9 and Q10 undertake a voltage comparison task, juxtaposing the reference voltage from the ZN423 with a segment of the regulator's output voltage.
This voltage slice derives from a potentiometer serving as the output voltage adjustment feature.
It's crucial to avoid substituting a zener diode for the ZN423, as the former's slope resistance reacts adversely to fluctuating battery voltages.
Adjustable Voltage Control and Pulse Width Modulation
As the potentiometer wiper voltage exceeds the reference voltage, Q8 activates.
Consequently, the ascending ramp at the base of Q6 must escalate to align with the voltage at the base of Q7.
This delay in activating the Darlington pair (Q4 and Q5) results in a narrower power output pulse to the choke.
This mechanism, known as pulse width modulation (PWM), in turn reduces the output voltage.
The nominal operating frequency is 20kHz, although it can vary considerably due to battery voltage fluctuations.
Output Stage and Energy Dissipation
The BY229-600 diode is specified for the catch diode, but in the prototype, a high-current rectifier proved effective.
The frequency of 20kHz seems manageable for these components.
Most wasted energy dissipates as heat in Q4, necessitating a suitable heat sink.
Output Voltage Feedback and Stability
The output voltage feedback attenuator incorporates 50Ω resistors to draw a minimum of 50mA from the output, essential for maintaining stability.
Alternatively, a 100Ω resistor could be shunted across the output, alongside a resistor and potentiometer (up to approximately 10kΩ each) to establish the correct feedback voltage.
When drawing 5A, a drop of 200mV in output voltage corresponds to an output resistance of 40 milliohms.
Grounding and Wiring
Designating the ground points with bold lines signifies their role as conduits for substantial currents.
These points necessitate robust connections, such as thick wiring or PCB tracks, tied back to a location proximate to the incoming battery's negative terminal.
This adherence to high-current grounding practices is a standard convention.
Output Regulation Strategies
The circuit does not currently encompass mechanisms for current limitation or crowbar protection.
Implementing a crowbar mechanism is straightforward, and current limiting can be achieved by manipulating Q9's base, thereby altering the power pulse's mark-space ratio.
Have Questions? Please Comment below to Solve your Queries! Comments must be Related to the above Topic!!